Topological Quantum Systems
The implications are far-reaching, from quasiparticles with nonlocal properties and nonabelian exchange statistics to novel approaches to quantum computing where topology may protect both storage and manipulation of quantum information.
In recent years, it has been realized that one can design materials with topological (p-wave) superconducting order by combining conventional superconductors and semiconductors with strong spin-orbit interaction. This exotic superconducting state with broken time-reversal symmetry is known to host Majorana modes which have been predicted to be both nonlocal and nonabelian. Nonlocal means that Majorana modes store quantum information in nonlocal degrees of freedom, implying that it cannot be read out or corrupted by local measurements or perturbations. Nonabelian means that quasiparticles exchange operations act non-trivially on the wavefunction and, in general, do not commute. These exotic properties are not only of fundamental interest but may also be exploited for protected storage and manipulation of quantum information in so-called topological quantum computing schemes.
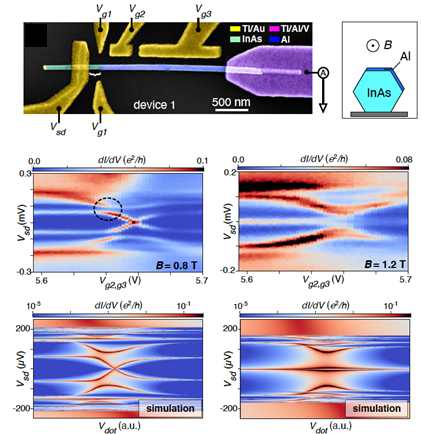
Transport experiments on a Majorana wire. The top shows a sketch of the nanowire with epitaxial Al together with a false color scanning electron micrograph of the device (blue: InAs nanowire with epitaxial Al, green: bare nanowire, yellow: gates, purple: bulk Al). The bottom panels show experimental data and corresponding theoretical simulations of the conductance of the device, which can be used to determine the spectrum of the hybrid nanowire close to the source contact. The figure is adapted from M. T. Deng et al., Science 354, 1557 (2016).
In the center, we focus on three different platforms of hybrid superconductor-semiconductor heterostructures, designed to support topological superconductivity, to allow experimental demonstration of nonlocal and nonabelian properties and to eventually become the basis for topological quantum computation. The semiconducting part is realized in the form of vapor-liquid-solid InAs nanowires, two-dimensional electron gases embedded in InAs/InGaAs heterostructures, and finally selective-area-growth InAs nanowires. All these materials are grown by means of molecular beam epitaxy (MBE) and directly contacted to an epitaxial Al layer in the MBE chamber without breaking the vacuum, thus guaranteeing the cleanest semiconductor/superconductor interface achievable nowadays.
We investigate the basic physics of these hybrid platforms by fabricating and measuring different types of devices, including, for example, normal-superconducting tunnel junctions, SQUIDs and Coulomb-blockaded superconducting islands. These measurements reveal important information about the properties of the hybrid platforms, such as spin-orbit coupling, spectrum of states below the superconducting gap, parity lifetime, etc.
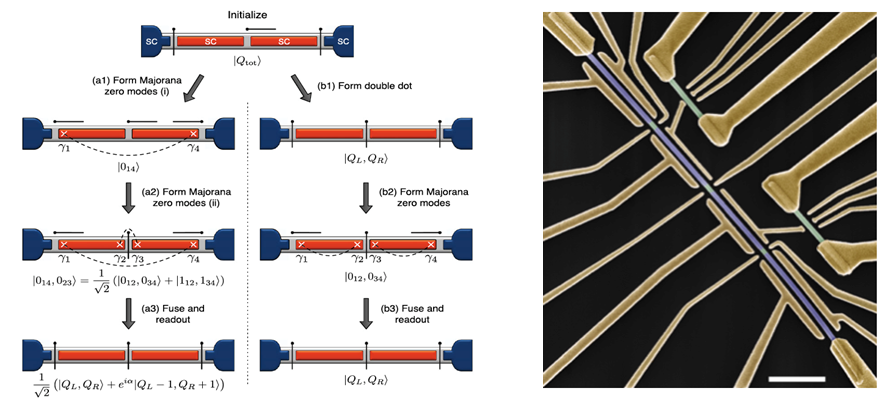
Left: Proposal (from D. Aasen et al., Phys. Rev. X 6, 031016 (2016)) for a device and a protocol to demonstrate the Majorana fusion rules, a property intimately connected with nonabelian exchange statistics. Right: False color scanning electron micrograph of a Majorana device for implementing the proposal in the left figure. The bare nanowires (green) are used as charge detectors which can read out QL and QR in the protocol in the left panel. The scale bar is 1 μm.
We also design and investigate sophisticated devices aiming to demonstrate the nonlocal and nonabelian properties of Majorana modes. Further down the road, these devices may form the basic building blocks in a future fault-tolerant topological quantum computer.
All activities involve a close collaboration between theory and experiments, which has proven crucial when translating abstract concepts from topology into actual devices and measurement protocols.